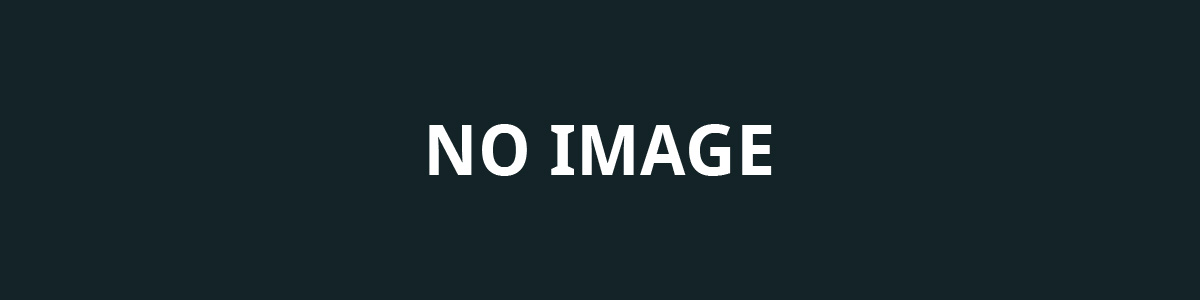
Understanding the Soil and Climate change
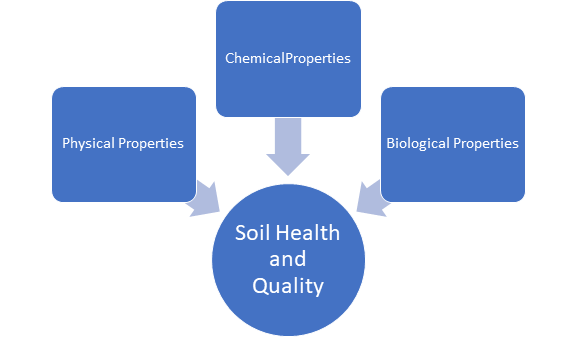
Properties of healthy and quality soil
Soil is simply defined as the medium for plants growth. The optimum growth of plants occurs on soils that are healthy enough to offer plants protection from different ailments and nutritional support. A healthy soil is the one that offers a combination of physical, chemical, and biological support processes. These characteristics help to promote ecological functions that are monitored in sustainable landscape management and climate change. Understanding the relationship between soil health and climate change is crucial for increased yields and, eventually, food security. https://extension.umaine.edu/cranberries/grower-services/workshops-and-meetings/berry-soil-management/
According to Allen et al. (2011), aggregate stability, soil organic matter (SOM), carbon and nitrogen cycling, microbial biomass and activity, as well as microbial fauna and flora variety are the common soil health markers that are affected by climate change. The impacts of global climate change drivers such as elevated temperature and CO2, varying precipitation, and atmospheric nitrogen are considered in the interaction.
The soil physical properties such as soil structure, porosity, infiltration, and bulk density affect some soil processes such as the availability of water in the soil that influence plant growth. Chemical soil properties such as pH, plant-available nutrients, salinity affect the soils by influencing the microbial activities and nutrients availability. The biological soil properties consider the microbial life of the soils that influence the decomposition of soil organic matter and then the availability of total soil carbon and nitrogen.
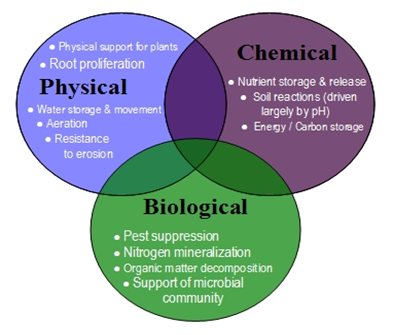
Soil types available in various agro-ecologies and altitudes differ in their properties and such variations make such agro-ecologies differ in their resilience to climate changes (Franke et al., 2018). These variations in combination with other local biophysical and social-economic conditions have been highlighted to be the cause of the varying adoption rates of soil fertility improvement practices under conservation agriculture (Waha et al., 2013, Müller et al., 2011). Mostly, these practices are universally promoted in the different agro-ecologies and their adoption has been reported lower than expected in most of the cases (Thierfelder et al., 2015).
The findings of the study I recently did on ‘modeling the impacts of integrating soybean (glycine max l.) in maize (Zea mays) cropping systems in the agro-ecological regions of Zambia’s show that soils containing fine particles like clay have the ability to support plant growth under relatively low moisture levels. Such higher yields resulted from the presence of a layer of clay accumulation that is existing within the rooting levels which contain high amounts of accessible nutritional ions such as calcium, magnesium, sodium, or potassium that support the growth of maize (Krogh and Greve, 1999). Clay has a relatively higher capacity of retaining soil organic compounds due to the larger surface area of its colloids that carry binding charges, thereby chemically stabilizing organic materials. This characteristic is different in soils that have a larger proportion of course particles.
Management practices on improving soils with higher coarse particles include reduced soil disturbance and maintenance of the above-ground crop residues. These practices should be promoted to ensure that such soils with higher course particle content have higher soil organic matter at the surface and enhance the improvement of the soil properties which make plants resilient to climate change impacts. Such being the case, precise and localized information on soil types and the associated climate change consequences may guide smallholder farmers in planning better farm interventions to adapt to their region-specific effects of climate change.
References
ALLEN, D. E., SINGH, B. P. & DALAL, R. C. Soil Health Indicators Under Climate Change: A Review of Current Knowledge. Soil Biology, 25.
FRANKE, A., VAN DEN BRAND, G., VANLAUWE, B. & GILLER, K. 2018. Sustainable intensification through rotations with grain legumes in Sub-Saharan Africa: A review. Agriculture, ecosystems & environment, 261, 172-185.
KROGH, L. & GREVE, M. 1999. Evaluation of World Reference Base for Soil Resources and FAO Soil Map of the World using nationwide grid soil data from Denmark. Soil Use and Management, 15, 157-166.
MÜLLER, C., CRAMER, W., HARE, W. L. & LOTZE-CAMPEN, H. 2011. Climate change risks for African agriculture. Proceedings of the National Academy of Sciences, 108, 4313-4315.
THIERFELDER, C., RUSINAMHODZI, L., NGWIRA, A. R., MUPANGWA, W., NYAGUMBO, I., KASSIE, G. T. & CAIRNS, J. E. 2015. Conservation agriculture in Southern Africa: Advances in knowledge. Renewable Agriculture and Food Systems, 30, 328-348.
WAHA, K., MÜLLER, C., BONDEAU, A., DIETRICH, J. P., KURUKULASURIYA, P., HEINKE, J. & LOTZE-CAMPEN, H. 2013. Adaptation to climate change through the choice of cropping system and sowing date in sub-Saharan Africa. Global Environmental Change, 23, 130-143.