Introduction
From my brief experiences in a university research laboratory, I became aware of the ability of a busy and highly productive biomolecular lab to generate a lot of normal and hazardous waste, use a lot of energy and are not actually working in a sustainable manner. After researching this topic, I concluded this could be said for most biomolecular labs. In terms of resource needs, energy usage, and trash output, research laboratories have a significant influence on the environment. Single-use plastic is also a hot topic these days, with global initiatives underway to clean up the seas of plastic debris. However, many analytical techniques can not rely on recycled plastic for specificity and contamination reasons. Plastics which are better for the environment while still being of a high-quality standard are starting to be seen in the form of Bioplastics.
Disruption of leachables from disposable plasticware
In biomolecular laboratories all over the world, disposable plastic ware is used. While much of this plastic is branded as “sterile,” providing researchers with some confidence that the products are free of bioactive contaminants, we now know that processing additives are unavoidable. Yet these chemical substances leaching out of plastic consumables or ‘leachables’ are still underestimated in the majority of life sciences applications. From carrying out my 4th year research project in a biomolecular lab I became very aware of how laboratory experiments can be influenced by various factors. From accidentally adding a fraction of a µL to much or too less, to forgetting to work under the fume hood I learned that a laboratory workplace is a very sterile place with little room for error. Contaminants that are bioactive Leaching out of consumables is often overlooked as a critical factor that can affect experimental results. Leachables are thought to have a variety of specific and general biological effects, making them a potential source of error in many assay systems (Rafal Grzeskowiak, 2015). The well-known company Eppendorf is a leading life science company that develops and sells instruments, consumables, and other services to laboratories worldwide. In a paper published by them in 2015 which can be accessed here ‘https://bit.ly/34yBg9L’ they describe leachables as chemical compounds that can be released into a sample from a given consumable under specific laboratory conditions. The effects of leachables are particularly relevant for plate-based assays, where a lot of variation in leachable levels have been observed in different wells, suggesting position dependant influences on the assay. Polymerase chain reaction (PCR) is a common tool in most biomolecular labs. PCR is used for the in vitro enzymatic amplification specific segments of DNA and it is a very popular tool for detecting rare sequences. DNA contamination from any sort of leachables can interfere with results or interpretation of sensitive assay analysis. Most of the consumables we would find in a laboratory consist of polymers manufactured from petrochemical-based monomers, most notably are polypropylene, polyethylene and polystyrene.
Rafal et al explained how contrary to belief most of the plastic ware we use in our laboratory’s contain various polymerization by-products beside the pure polymer. Various chemicals are added to reduce production costs but ultimately alter the consumable properties. With the overdue agenda of reducing single use plastic in laboratories will the high standard for plastic ware from the likes of Eppendorf be reduced and will there be a problem with using recycled plastic for PCR reactions. PCR analysis need to reach very high temperatures for denaturing, temperatures can reach up to 95˚C during this stage. Single use plastic that is used for such PCR reactions have to be able to not only withstand these high temperatures but to be able to heat and cool over a long period. It is unlikely that recycled plastic would be used in these sensitive assay analyses as results could be sabotaged.
Rafal Grezeskowiak carried out a study where he analysed using HPLC (High Performance Liquid Chromatography), UHPLC (Ultra High Pressure Liquid Chromatography) , GC (Gas Chromatography) and MS (Mass Spectrometry) methods the organic substances leaching from standard microcentrifuge tubes from different manufacturers into samples after incubating under typical assay conditions with water. He was able to identify high amounts of organic substances migrating into samples, Fig1 shows the identified polypropylene additives including nucleating agents and antioxidants.
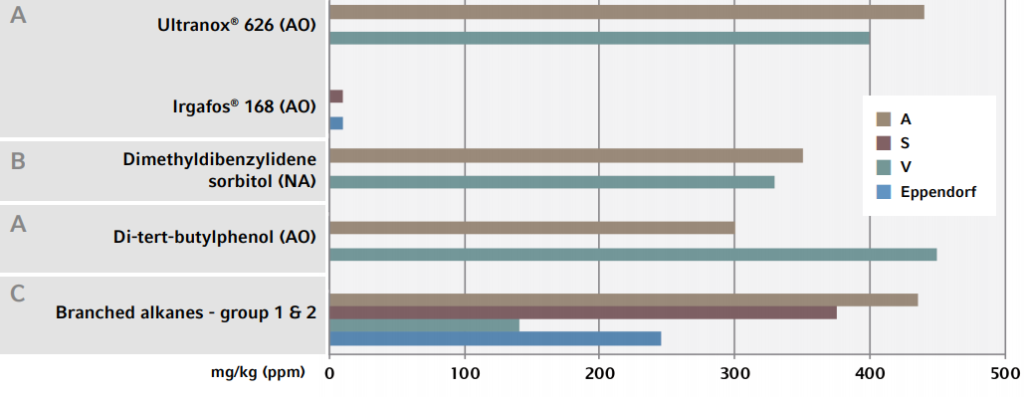
Fig 1. Identification and quantification of extractables in samples incubated in microcentrifuge tubes.(Rafal Grzeskowiak, 2021).
So, should we consider what the possibilities are to produce lab-grade plastics from recycled material? When dealing with recycled plastics it can be very difficult to determine the precise origin of the material or the contaminants that may be present. In William van Grunsven article he highlights how producers put a lot of effort into purifying plastics during the recycling process, however the purity of the recycled material is much lower than virgin plastics (Hopewell et al., 2009).
Disposable plastics are thought to be more practical and cost effective, because of the nature of toxicity of laboratory plastics, they have long been considered non-recyclable. The current practice of most labs for contaminated cell culture plastics are to bag, autoclave and send them to the land fill. Even plastic test tubes which have only held water are disposed of this way as recycling plants see them as being a health and safety concern(Kuntin, 2018). Many researchers are enthusiastic about recycling single-use plastics, but we are surrounded by disposable plastic in the conventional biomolecular laboratory, which is often not recycled due to biological pollution. There have been many innovations to try and combat this issue, one such idea I found interesting was invented by David Kuntin, a University of York researcher. David made a ‘decontamination station’, a container that allowed for a 16-hour soak in a high-level disinfectant followed by a water rinse for chemical decontamination, as a way to recycle tissue culture flasks (Kuntin, 2018).
For a laboratory to aim to be more sustainable it isn’t always as straight forward as reducing or reusing the plastic ware use in the laboratory. Plastic Petri dishes do not retain their form when autoclaved at high temperatures, making it impossible to reuse them without risking contamination(Alves et al., 2021).Often glass pieces can be decontaminated by autoclaving and reused without the use of a chemical decontamination station. When centrifuging samples glass culture may not be suitable, and when conducting tissue culture procedures that require non-pyrogenic and non-cytotoxic materials reusable glass substitutes will not be able to meet these requirements.
A Sustainable-Lab Consumables Guide has been developed by University College London, which highlights all of the improvements that research laboratories may make to become more sustainable, you can find this guide here Sustainable Lab Consumables Guide | Sustainable UCL – UCL – University College London.
How to use plastic more sustainable in the lab
As researchers we need to become better informed about what plastics we can use which hinder experiments but are also environmentally friendly. We need to become aware of the most commonly recycled plastics which are polystyrene (PS), polypropylene (PP) and high density or low density polyethylene (HDPE/LDPE). PP is used for popular consumables like centrifuge tubes, while PS is used for culture dishes and flasks. Lids are most often made of HDPE and LDPE (Kuntin, 2018). An interesting programme called LEAF (Laboratory Efficiency Assessment Framework) has been developed at University Collage London (UCL) to improve the sustainability and efficiency of laboratories. This programme offers research laboratories a guide to save plastics, water, energy and other resources. UCL claim that by taking part in this programme laboratories ‘will reduce their carbon emissions and create an environment that supports research quality’. Laboratories then receive a Gold, Silver or Bronze award depending on their sustainability actions. You can find out more information on this programme here https://bit.ly/32CF5d7 .
Problems with single use plastic
with an estimated 20,500 institutions worldwide involved in biological, medical or agricultural research, Urbina et al estimated that in 2014 these institutes likely generated around 5.5 million tonnes of lab plastic waste in 2014(Urbina et al., 2015). This would be the equivalent of 67 cruise liners and 83 percent of all plastic recycled globally in 2012. Single-use plastics (SUPs) are materials designed to be used once and then discarded. In a laboratory, single-use plastic can range from packaging to syringes to beakers. SUPs has many benefits in the lab: it is less expensive, easier to standardise, and often arrives sterile before being used and discarded. SUPs have also been shown to degrade slowly and fracture into small pieces with diameters less than 5 mm, referred to as secondary microplastics (MPs), when exposed to UV rays, heat, or mechanical stress(Chen et al., 2021). Plasticizers such as phthalates or phthalic acid esters (PAEs) are commonly used in SUP products to improve the plasticity of resin molecules, making the plastic more flexible and simple to process(Chen et al., 2021). These PAEs can leach into the natural environment and even enter the public food chain. In Turkey PAEs were detected in four popular drinks (soda, lemonade, cola and mineral water) (Ustun et al., 2015).
Bioplastics
According to European Bioplastics, bioplastics are polymers that are generated from renewable sources and biological systems (bio-based) and/or are biodegradable(Samantaray et al., 2020). Bioplastics production is expected to attain a 40% share of the plastics industry by 2030, driven by a low-carbon circular economy (European-bioplastics, 2019). Development of biodegradable and bio-mass derived polymers to replace non-biodegradable and petrochemical-based plastics can be a sustainable step toward a low carbon footprint. Glycolic acid, a polyglycolic acid (PGA) monomer, is currently mostly used as a co-monomer in the production of poly(lactic-co-glycolic acid) (PLGA) copolymers to balance the mechanical strength and biodegradability of polylactic acid (PLA)(Samantaray et al., 2020).PLCA copolymers are a kind of biodegradable polymer recognised by the Food and Drug Administration (FDA) for use in biomedical applications, the use of PGA-based copolymers in fields such as shape memory films, antimicrobial coatings, food packaging goods, and biomedical scaffolds will expand the possibilities for manufacturing high-performance and useful green plastics(Samantaray et al., 2020). These bioplastics have the strength and means of replacing single use plastic in biomolecular laboratories. The bio-based and biodegradable plastic is a niche market with growing popularity, for instance sustainably cultivated seaweeds as feedstocks for biodegradable bioplastics (Helmes et al., 2018). In this project they were able to produce a PLA polymer from Kelp, a raw material for 3D printing, this has intrigued other EU countries into producing sustainable bioplastic (Hasselström et al., 2020). The concept of reducing, reusing and recycling is the framework around the circular economy, the use of bio-degradable plastics over single use plastics should be promoted and invested in by influential government and private bodies.
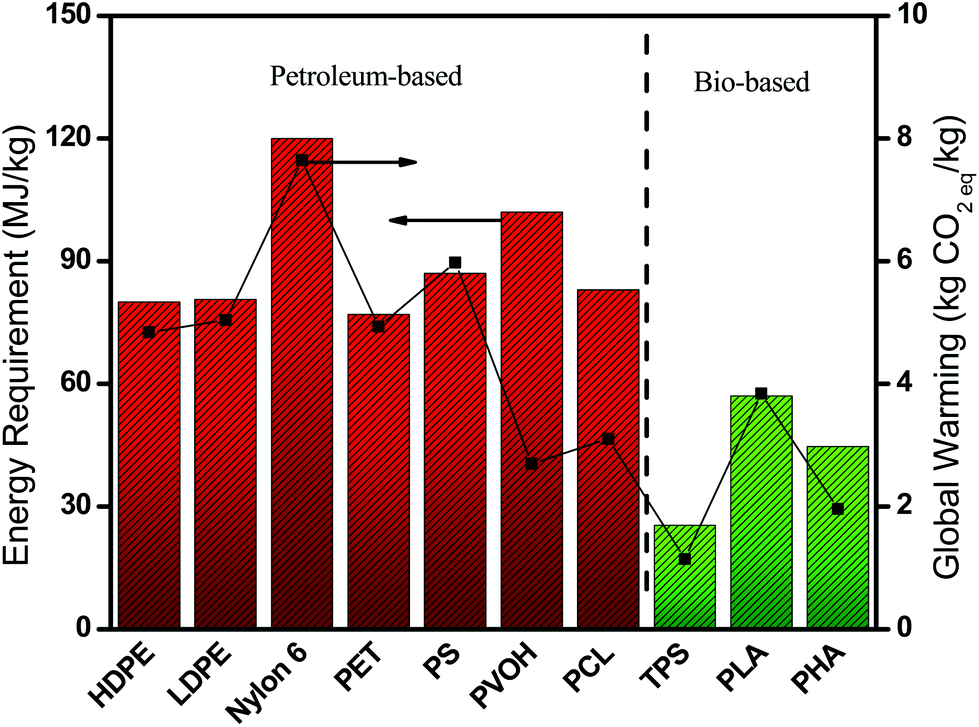
Fig 2. This figure compares the impact of Bio-based polymers on the environment compared to non-biodegradable petroleum-based polymers. (Meereboer et al., 2020).
Conclusion
As a concluding remark we as researchers need to think of the plastic we are using, I would like to encourage all research scientists, to consider how they might limit the quantity of single-use plastic objects used in their daily research. This obviously is not possible in all cases as recycled or bioplastic may not meet the standard of quality needed. Continued research and development into bio-based biodegradable polymers could be very beneficial to reducing the single use plastic in biomolecular laboratories and is also critical for a sustainable circular economy that mitigates the harmful effects of conventional plastics on the environment and eco-system.
References:
<Consumables_White-Paper_026_Consumables_Leachables-Minimizing-Influence-Plastic-Consumables-Laboratory-Workflows.pdf>.
ALVES, J., SARGISON, F. A., STAWARZ, H., FOX, W. B., HUETE, S. G., HASSAN, A., MCTEIR, B. & PICKERING, A. C. 2021. A case report: insights into reducing plastic waste in a microbiology laboratory. Access Microbiology, 3.
CHEN, Y., AWASTHI, A. K., WEI, F., TAN, Q. & LI, J. 2021. Single-use plastics: Production, usage, disposal, and adverse impacts. Sci Total Environ, 752, 141772.
EUROPEAN-BIOPLASTICS 2019. Bioplastics Market Development Update.
HASSELSTRÖM, L., THOMAS, J.-B., NORDSTRÖM, J., CERVIN, G., NYLUND, G. M., PAVIA, H. & GRÖNDAHL, F. 2020. Socioeconomic prospects of a seaweed bioeconomy in Sweden. Scientific Reports, 10, 1610.
HELMES, R. J. K., LÓPEZ-CONTRERAS, A. M., BENOIT, M., ABREU, H., MAGUIRE, J., MOEJES, F. & BURG, S. W. K. V. D. 2018. Environmental Impacts of Experimental Production of Lactic Acid for Bioplastics from Ulva spp. Sustainability, 10, 2462.
HOPEWELL, J., DVORAK, R. & KOSIOR, E. 2009. Plastics recycling: challenges and opportunities. Philosophical transactions of the Royal Society of London. Series B, Biological sciences, 364, 2115-2126.
KUNTIN, D. 2018. How to reduce your lab’s plastic waste The Biologist
MEEREBOER, K. W., MISRA, M. & MOHANTY, A. K. 2020. Review of recent advances in the biodegradability of polyhydroxyalkanoate (PHA) bioplastics and their composites. Green Chemistry, 22, 5519-5558.
RAFAL GRZESKOWIAK 2015. Leachables: Minimizing the Influence
of Plastic Consumables on the Laboratory
Workflows. Eppendorf AG, Germany.
RAFAL GRZESKOWIAK 2021. Extractables and Leachables in
Microcentrifuge Tubes – Extensive
HPLC/GC/MS Analysis.
SAMANTARAY, P., LITTLE, A., HADDLETON, D., MCNALLY, T., TAN, B., SUN, Z., HUANG, W., JI, Y. & WAN, C. 2020. Poly (glycolic acid) (PGA): a versatile building block expanding high performance and sustainable bioplastic applications. Green Chemistry, 22.
URBINA, M. A., WATTS, A. J. R. & REARDON, E. E. 2015. Labs should cut plastic waste too. Nature, 528, 479-479.
USTUN, I., SUNGUR, S., OKUR, R., SUMBUL, A. T., OKTAR, S., YİLMAZ, N. & GOKCE, C. 2015. Determination of phthalates migrating from plastic containers into beverages. Food Analytical Methods, 8, 222-228.